PACE-CME
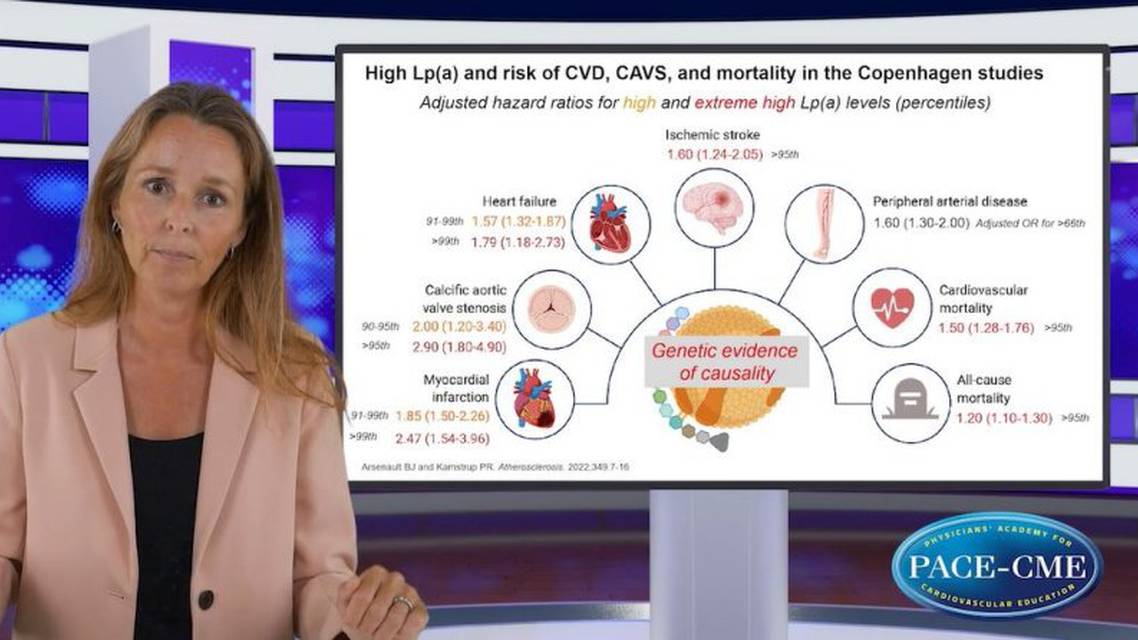
Subtitles available: English, French, German & more
Targeting Lp(a) in CV risk reduction: where are we now?
Welcome to this lecture entitled: Targeting Lp(a) in Cardiovascular Risk Reduction: Where are we now? I am Pia Kamstrup from the Copenhagen General Population Study and the Department of Clinical Biochemistry Copenhagen University Hospital Herlev Gentofte in Denmark. These are my disclosures.
Now Lp(a) is produced by the liver and consists of an cholesterol-laden LDL-like particle covalently bound to a large plasminogen-like glycoprotein apolipoprotein(a). Plasminogen contains five different types of so called kringle structures as well as a protease domain. While the apolipoprotein(a) only contains several different types of kringle IV structures, namely, type 1 through 10, as well as a kringle 5 structure and an inactive protease domain. Importantly, the number of kringle IV type 2 structures vary between 2 and more than 40, giving rise to the apolipoprotein(a) isoform size variation.
Levels of Lp(a) are primarily genetically determined by variation in the LPA gene, but vary substantially between individuals as shown here on the concentration distribution based on a large general population sample from Copenhagen, Denmark. Now the concentration distribution is highly skewed with the long tail stretching out towards high and extremely high levels, and approximately 20% of the population is found in the tail part of the concentration distribution. It is well known that both the concentration distribution as well as median levels do vary with ethnicity. Thus, individuals of African descent, for example, usually have a less skewed concentration distribution and higher levels. Nonetheless, plasma levels are 80% to 90% genetically determined by variation in the LPA gene, including the kringle IV type 2 copy number variant determining apolipoprotein(a) size, which correlates inversely with plasma Lp(a) levels.
In 1963, Lp(a) was first described as a distinct beta lipoprotein by the Norwegian Kohlberg. In 1987 Lon and colleagues cloned the LPA gene discovering the remarkable similarity to plasminogen prompting research into also possible prothrombotic effects of Lp(a) in addition to pro-atherosclerotic effects. However, in 1991, the first prospective studies were published, yielding no findings. This led to a severely dampened interest in the Lp(a) research field, also illustrated by the dip in Lp(a) related publications in the years following the early '90s. In 2009, three key publications resurrected the Lp(a) field, so to speak. First, a large meta-analysis by the emerging risk factors collaboration, combining individual participant data on more than 100,000 participants, and showing curvilinear associations of Lp(a) levels with risk of non-fatal MI and coronary death, and also for the highest levels increased risk of ischemic stroke. Two large genetic studies then subsequently provided genetic evidence of causality. The PROCARDIS study where the LPA gene was found to have the strongest association with coronary heart disease out of 2,100 variants examined. Specifically, two single nucleotide polymorphisms in the LPA gene were strongly associated with plasma Lp(a) levels, as well as with risk of coronary disease. Also in a classic mendelian randomization study, as shown here on the right part of the slide, a stepwise increase in risk of myocardial infarction was found with increasing levels of Lp(a). Levels above the 90th percentile predicted two to three fold increases in risk, but with risk already starting to increase at more moderate levels. The study provided a strong genetic evidence of causality as seen in the bottom part of the slide, where number of kringle IV type 2 repeats, also associated with plasma Lp(a) levels and with risk of myocardial infarction in the expected direction. These two genetic studies give strong evidence of causality as genotype disease associations are generally not prone to confounding and may not resolve from reverse causation, as disease cannot alter genotypes determined at conception.
Now looking at stratified analysis in all three of the studies, it was also demonstrated that the presence of other known risk factors, such as high age, male sex, hypertension, diabetes, or elevated LDL cholesterol, did not significantly influence the risk of coronary heart disease associated with high Lp(a) or corresponding LPA risk genotypes. Thus, the LPA-associated risk appeared entirely independent of other risk factors.
More recently, similar strong evidence of a causal association of high Lp(a) levels with risk of calcific aortic valve disease stenosis has been provided. First, in a landmark meta-analysis of GWAS data on 7,000 individuals where the LPA gene was strongly implicated in aortic valve calcification, as shown here on the Manhattan plot, top left. In subsequent studies, a stepwise increase in risk of aortic valve stenosis was seen with increasing levels of Lp(a) as seen on the bottom, and where levels above the 90th percentile as for myocardial infarction predicted two to threefold increases in risk. Finally, in aortic stenosis patients, Lp(a) and pro-inflammatory oxidized phospholipids associated with progressive valve calcification and disease.
While the highest risk estimates have been reported for myocardial infarction and calcific aortic valve stenosis, high Lp(a) levels have also been found to associate with increased risk of heart failure, ischemic stroke, lower peripheral arterial disease, and cardiovascular and all-cause mortality. Importantly, for all endpoints, genetic evidence of causality exists as LPA risk genotypes associated with high plasma Lp(a) levels, likewise associates with each endpoint.
Further genetic evidence of causality has been provided from studies examining LPA genetic variants associated with low Lp(a) levels. For example, in a very large genetic study including more than 100,000 participants, a gene risk score based on four LPA SNPs associated with low Lp(a) levels, associated with decreased risk of cardiometabolic disease, including stroke, coronary heart disease, aortic valve stenosis, heart failure, and peripheral vascular disease.
While most data on Lp(a) associated risk stemmed from primary prevention populations, some data in secondary prevention populations also excluding data from post-hoc analyses of cardiovascular outcome trials targeting LDL cholesterols does exist. Thus, in the Copenhagen General Population Study, it was recently demonstrated that increasing levels of Lp(a) associate with a stepwise increase in risk of recurrent major adverse cardiovascular events as shown on this slide, and with a twofold increase in risk found for the very highest levels.
Finally, while most data also stemmed from White or European descent populations, in a recent large study from the UK Biobank including more than 450,000 individuals, a significant number of self-identified South Asian and Black individuals as well as a smaller number of Chinese individuals were included. On the left part of the slide, you see that there are the expected significant differences in Lp(a) levels according to race ethnicity. However, a similar log-linear rise in risk of ASCVD was found with increasing Lp(a) levels across racial ethnic subgroups with a hazard ratio of 1.11 per 50 nmol/L increase in plasma Lp(a) levels, and with risk already starting to increase at more moderate levels that is just above the median and, say, at 30 milligrams per deciliter. Additionally, it was shown that using a race-specific 90th percentile, similar risk estimates were also found for increased Lp(a) levels, and some evidence that a uniform threshold of 150 nmol/L may be applicable across different race ethnicities was also found as shown here also, bottom right.
While we await results from cardiovascular outcome trials, reassuringly large genetic epidemiologic studies have recently demonstrated that the only observationally and genetically concordant association found for low plasma levels of Lp(a) and corresponding LPA genotypes was with decreased risk of diseases of the circulatory system as expected. No concordant association was found with any other major disease groups. These results thus indicate that lowering Lp(a) levels will likely be safe.
Now summing up key take aways from this lecture. High Lp(a) levels are common and primarily genetically determined. Cardiovascular risk increases at Lp(a) levels above 30 milligrams per deciliter and even more at levels above 50 milligrams per deciliter. Lp(a) levels in the top 10% of the concentration distribution, roughly corresponding to levels above 70 milligrams per deciliter or above 115 nanomoles per liter predict two to threefold increases in risk of coronary heart disease and calcific aortic valve stenosis. Also, Lp(a) associated risk is independent of other risk factors and there exists strong genetic evidence of causality. Presently, we eagerly await results from cardiovascular outcome trials to provide final evidence of causality for high Lp(a) levels.
Recommended
- Targeting Lp(a): therapeutic insights and novel developmentsTargeting Lp(a): therapeutic insights and novel developmentsLp(a): from causality to therapyTargeting Lp(a): therapeutic insights and novel developments
- Lp(a) as independent causal risk factor: why should we focus on testing?Lp(a) as independent causal risk factor: why should we focus on testing?Lp(a): from causality to therapyLp(a) as independent causal risk factor: why should we focus on testing?